Plenary Lectures
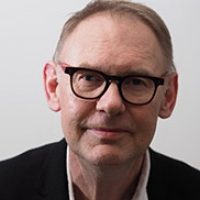
Ian R. Petersen was born in Victoria, Australia. He received a Ph.D in Electrical Engineering in 1984 from the University of Rochester. From 1983 to 1985 he was a Postdoctoral Fellow at the Australian National University. From 2017 he has been a Professor at the Australian National University. He was the Interim Director of the Research School of Electrical, Energy and Materials Engineering at the Australian National University from 2018-2019. From 1985 until 2016 he was with UNSW Canberra where he was a Scientia Professor and an Australian Research Council Laureate Fellow in the School of Engineering and Information Technology. He has previously been ARC Executive Director for Mathematics Information and Communications, Acting Deputy Vice-Chancellor Research for UNSW and an Australian Federation Fellow. He has served as an Associate Editor for the IEEE Transactions on Automatic Control, Systems and Control Letters, Automatica, IEEE Transactions on Control Systems Technology and SIAM Journal on Control and Optimization. Currently he is an Editor for Automatica. He is a fellow of IFAC, the IEEE and the Australian Academy of Science. His main research interests are in robust control theory, quantum control theory and stochastic control theory.
Using Systems and Control Theory Ideas in the Design of Quantum Amplifiers
One of the most significant areas emerging in the area of quantum technology is that of quantum computing. Companies such as Google, IBM, and Microsoft have made significant investments in quantum computing to develop small scale quantum computers using microwave frequency technologies involving arrays of superconducting Josephson junctions operating at millikelvin temperatures. Other technologies which have been investigated for the implementation of quantum computers include quantum optics, ion trap devices and solid state quantum technologies. Quantum amplifiers play a critical role in many of these quantum computing technologies in that they are required to read out qubit states and transfer the information to the classical world.
Quantum amplifiers are examples of linear quantum systems and can be analysed using the recently developed theory of quantum linear systems. We begin with an introduction to quantum linear systems theory including the concept of physical realizability. We then present a systems theory approach to the design of quantum amplifiers minimizing the amount of quantum noise introduced by the amplifier whilst still guaranteeing desired properties of the amplifier such as the phase-insensitive property and the non-reciprocal property. We also consider the achievable gain and bandwidth of quantum amplifiers. These methods can be applied to amplifiers implemented using a quantum optics technology or a superconducting microwave technology. Our approach is based on a singularly perturbed quantum system involving the broadband approximation of a Bogoliubov transformation. In the case the optical implementation of a phase-insensitive amplifier it requires two squeezers and two beamsplitters. In the case of the optical implementation of a non-reciprocal and phase-insensitive quantum amplifier it requires three squeezers and two beamsplitters.
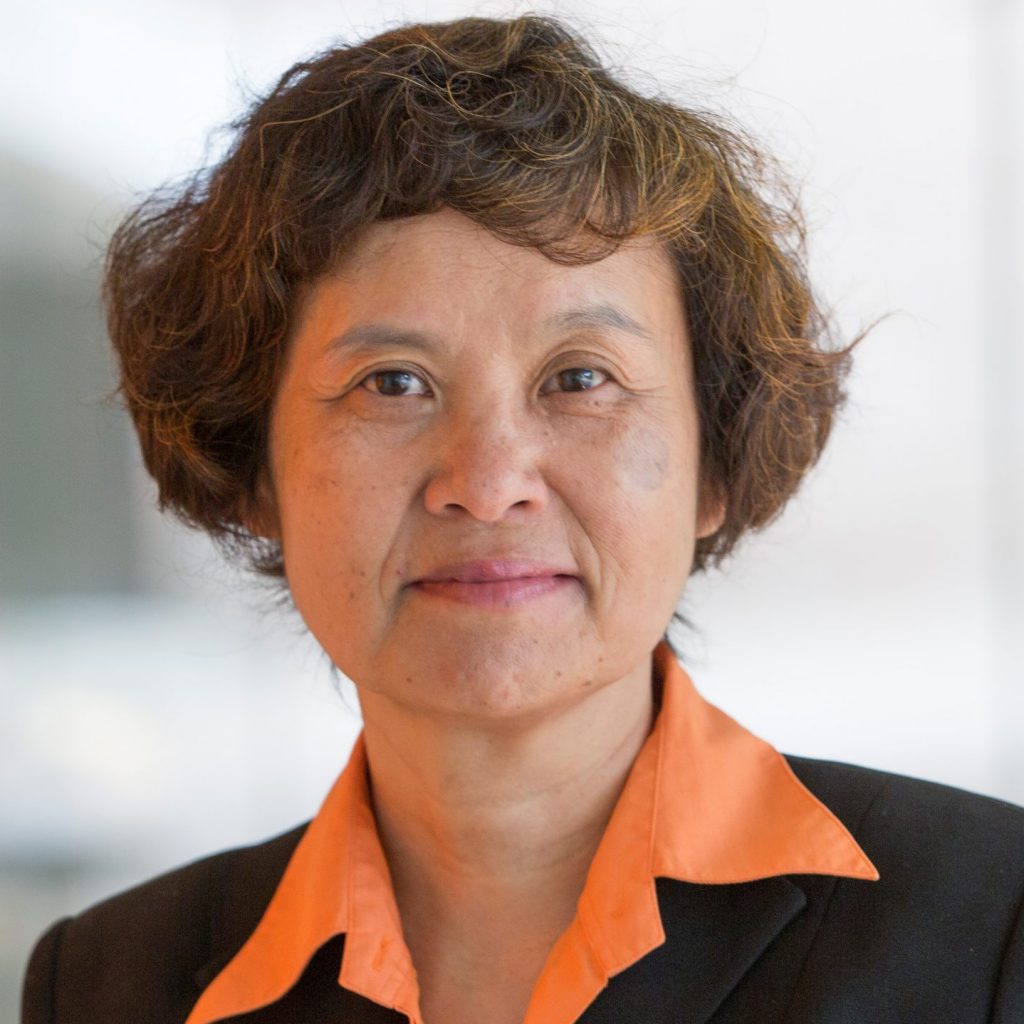
Jing Sun received her Ph. D degree from the University of Southern California in 1989 and her master’s and bachelor’s degrees from the University of Science and Technology of China in 1984 and 1982. From 1989-1993, she was an assistant professor in the Electrical and Computer Engineering Department at Wayne State University. She joined Ford Research Laboratory in 1993, where she worked on advanced powertrain system controls. After spending almost ten years in the industry, she returned to academia in 2003. She joined the University of Michigan, where she is the Michael G. Parsons Collegiate Professor and the chair in the Naval Architecture and Marine Engineering Department. She holds joint appointments in the Electrical Engineering and Computer Science Department and Mechanical Engineering Department at the same university. She has been named the inventor of 41 US patents and has published over 300 archived journal and conference papers. She is a fellow NAI (the National Academy of Inventors), IEEE, IFAC, and SNAME (the Society of Naval Architecture and Marine Engineering). She is one of the three recipients of the 2003 IEEE Control System Technology Award.
Prediction, Estimation, and Control of Connected and Autonomous Vehicles
Connected and Automated Vehicles (CAV) have been heralded as the transformative technology, leading us to the new era of transportation with unprecedented safety and mobility benefits. They also push the energy efficiency of the transportation systems at both the macro and micro (vehicle) levels to the next height with abundant new opportunities for communication and optimization. While advanced sensors and hardware, such as camera, radar, and lidar and those used in V2V and V2I communications, have been featured predominantly in CAV showcases, control again is playing the role of the “unsung” hero that enables the CAV technology in the “hidden” world with algorithms and computational intelligence. In this talk, we will discuss some fundamental technical challenges for prediction, estimation, and control at the core of the CAV technology. Using the integrated power and thermal management for CAV as an example, we will show how model-based design, complemented by data-driven approaches, has led to control and optimization solutions with a significant impact on energy efficiency and operational reliability. Open problems will be highlighted to stimulate interest from our community to sing our song loudly and collectively on this CAV platform.
Transition to Practice Award Lecture
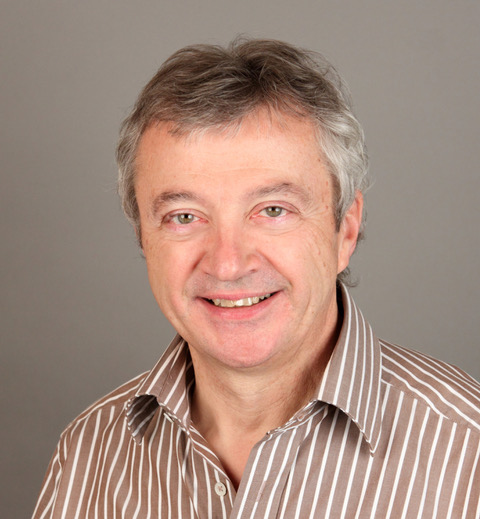
Guy A. Dumont received the Dipl. Ing. degree from Ecole Nationale Supérieure d’Arts et Metiers, Paris, France, in 1973, and the Ph.D. degree in electrical engineering from McGill University, Montreal, QC, Canada, in 1977. He was with Tioxide, France, from 1973 to 1974, and again from 1977 to 1979. He was with Paprican from 1979 to 1989, first in Montreal and then in Vancouver. In 1989, he joined the Department of Electrical and Computer Engineering, University of British Columbia, where he is a Professor and Distinguished University Scholar. From 2000 to 2002, he was the Associate Dean, Research for the Faculty of Applied Science Since 2008 he has been an Associate Member of the UBC Department of Anesthesiology Pharmacology and Therapeutics. He also is a Principal Investigator at the BC Children’s Hospital Research Institute and co-founder and co-Director of the Digital Health Innovation Laboratory (DHIL).
His current research interests include patient monitoring; signal processing for physiological monitoring; physiological closed-loop control systems such as automated drug delivery in anesthesia; circadian rhythms; global and mobile health; non-contact patient vital sign assessment; and brain monitoring via electroencephalography and near-infrared spectrometry.
Dr. Dumont was awarded a 1979 IEEE Transactions on Automatic Control Honorable Paper Award; a 1985 Paprican Presidential Citation; a 1990 UBC Killam Research Prize; the 1995 CPPA Weldon Medal; the 1998 Universal Dynamics Prize for Leadership in Process Control Technology; the IEEE Control Systems Society 1998 Control Systems Technology Award; three NSERC Synergy Awards, the latest one in 2016 for the development of the Phone Oximeter; the 2010 Brockhouse Canada Prize for Interdisciplinary Research in Science and Engineering. In 2011–12, and again in 2018-19, he was a UBC Peter Wall Distinguished Scholar in Residence. In 2020 he was awarded the IEEE Control Systems Society Transition to Practice Award. He has been a Fellow of the IEEE since 1998, and in 2017 he was elected a Fellow of the International Federation of Automatic Control as well as a Fellow of the Royal Society of Canada.
From rotary kilns and paper machines to anesthesia and COVID-19: the broad reach of control engineering
Karl Aström once famously called automatic control the hidden technology in recognition of the fact that despite its pervasiveness, it is rarely mentioned. Control is indeed a critical component of so many technologies used in industry and in our everyday life. In this talk I want to illustrate the broad reach of control engineering through applications I performed over the last forty years. In the process industries, I have developed and implemented adaptive control of rotary kilns for the production of TiO2 pigments, robust cross-directional control of paper machines, adaptive control of continuous digesters, dual adaptive control of wood-chip refiners and paper coating. I have developed a general-purpose adaptive controller based on Laguerre functions, that has been applied to the control of processes for the production of lime, glass, oil and gas, food and beverage. For the last two decades, working in close collaboration with clinicians I have focused on biomedical applications especially in critical care and automated intravenous anesthesia. Over the last year, COVID-19 has presented a challenge to the public health authorities. By representing control of COVID-19 explicitly in a feedback framework, transparent and effective public health policies can be systematically designed and rigorously analyzed, replacing ad-hoc policies. This requires us to work closely with epidemiologists and public health experts. Through this talk I want to illustrate the breadth of control applications and the sense of excitement a career in control can bring particularly when working in a multidisciplinary environment. I have been at it for some 45 years and still feel as passionate about my work as when I started, and I hope to convey this passion to our younger colleagues. We bring a unique perspective and have much to contribute to society at large, it is time to come out of hiding!
Keynote Lectures
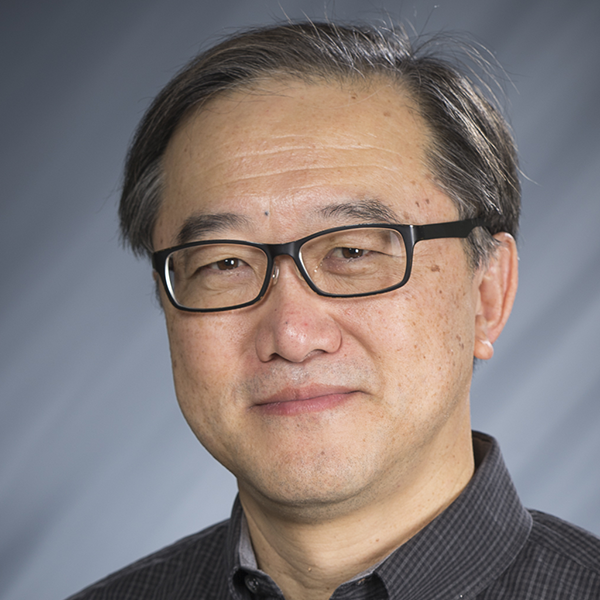
George Chiu is a Professor in the School of Mechanical Engineering with courtesy appointments in the School of Electrical and Computer Engineering and the Department of Psychological Sciences at Purdue University. He also has a half time appointment as the Assistant Dean for Global Engineering Programs and Partnerships in the College of Engineering. Chiu received the B.S. degree in Mechanical Engineering from National Taiwan University in 1985 and the M.S. and Ph.D. degrees from the University of California at Berkeley, in 1990 and 1994, respectively. Before joining Purdue, he worked for the Hewlett-Packard Company, designing inkjet printer and multi-function devices. From 2011-14, he served as the Program Director for the Control Systems Program in the Engineering Directorate of the National Science Foundation. His current research interests are mechatronics and dynamic systems and control with applications to digital printing and imaging systems, digital fabrications, human motor control and robotics, motion and vibration perception and control. He received the 2012 NSF Director’s Collaboration Award, the 2010 IEEE Transactions on Control System Technology Outstanding Paper Award and the Purdue University College of Engineering 2010 Faculty Engagement/Service Excellence Award and 2006 Team Excellence Award. Dr. Chiu served as the Editor-in-Chief for the IEEE/ASME Transactions on Mechatronics from 2017-19 and as the Editor for the Journal of Imaging Science and Technology from 2012-14. He is a Fellow of ASME and the Society for Imaging Science and Technology (IS&T) and a senior member of IEEE.
Modeling and Control of Digital Printing and Imaging Systems
Digital printing and imaging systems are well-recognized mechatronics devices. They are an integral part of our daily lives. Although traditional print media has been in decline, recent interests in leveraging printing as a scalable fabrication/manufacturing process has renewed the development of functional printing as an additive manufacturing process. In addition to deposit precisely controlled amount of material with the necessary spatial accuracy, interaction between material and substrate as well as material with themselves all contribute to the geometry, functionality and quality of the final product, be it an image, device or structure. In this talk, I will present our experiences in applying different mechatronic techniques to several digital printing and imaging processes as well as sharing some insights gained when translating these approaches to other applications with similar implementation and real-world constraints.
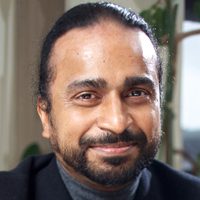
Santosh Devasia received the B.Tech. (Hons) from the Indian Institute of Technology, Kharagpur, India, in 1988, and the M.S. and Ph.D. degrees in Mechanical Engineering (ME) from the University of California at Santa Barbara in 1990 and 1993 respectively. He is the Director of the Boeing Advanced Research Center (BARC) at the University of Washington (UW) https://depts.washington.edu/barc/ and the faculty lead of the new Advanced Composite Center aimed at robotic manufacturing methods for emerging recyclable thermoplastic composites. He is the Nabtesco Professor of Engineering at the UW, Seattle. He joined the faculty of the UW Mechanical Engineering (ME) Department in 2000 after teaching from 1994 to 2000 in the ME Department at the University of Utah, Salt Lake City. He served as the Associate Chair of the UW ME Department at UW from 2010-2013, and as the Associate Dean of Research and Faculty Affairs in the College of Engineering at UW from 2013-2017. He was the General Chair for the 2020 American Control Conference and will chair the 2023 Advanced Intelligent Mechatronics Conference in Seattle, WA. He is a fellow of ASME and IEEE. His current research interests include control of multi-agent systems and precision human-machine systems. Additional details of current efforts can be found at: http://faculty.washington.edu/devasia/
Cohesive networks using delayed self-reinforcement
How a decentralized network gets to the goal (a consensus value) can be as important as reaching the consensus value. While prior methods focus on rapidly getting to a new consensus value, maintaining cohesion, during the transition between consensus values or during tracking, remains challenging and has not been addressed. Maintaining cohesion is important, e.g., to maintain inter-vehicle spacing in connected automated transportation systems, alignment synchronization to help maintain formations during maneuvers of flocks and swarms in nature, to avoid damage due to large deformations when transporting flexible objects and to maintain formation of engineered networks such as satellites, unmanned autonomous vehicles and collaborative robots.
The challenge to maintain cohesion arises because information about the desired response (such as the desired orientation or speed of the agents) might be available to only a few agents in a decentralized framework. The desired-response information needs to be propagated through the network to other agents, which results in response-time delays between agents that are “close to” the information source and those that are “farther away.” The talk will present a delayed self-reinforcement (DSR) approach, where each individual augments its neighbor-based information update using its previously available updates, to improve cohesiveness of the response during transitions. The advantages of the proposed DSR approach are that it only requires already-available information from a given network to improve the cohesion and does not require network-connectivity modifications (which might not be always feasible) nor increases in the system’s overall response speed (which can require larger input). Results are presented that show substantial improvement in cohesion with DSR.
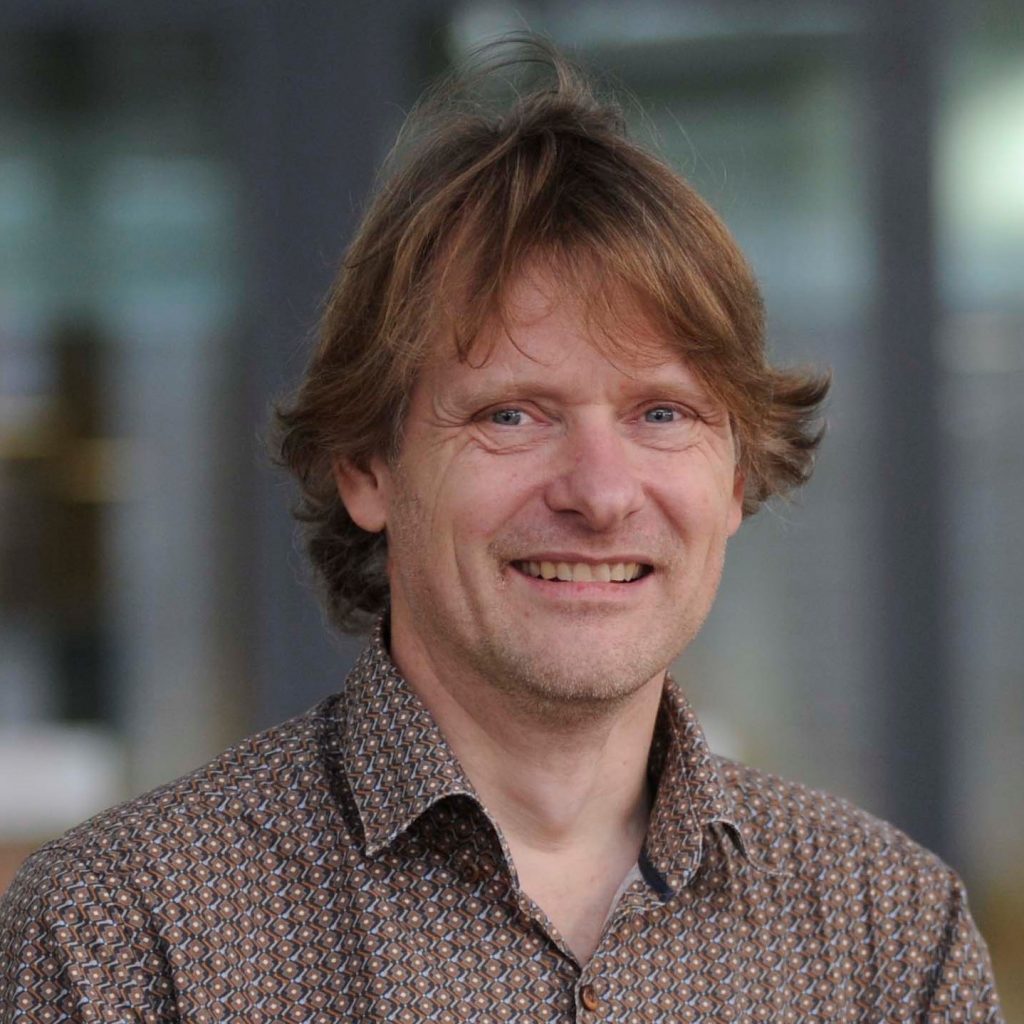
Marcel Heertjes received the M.Sc. and Ph.D. degrees from the Eindhoven University of Technology, Eindhoven, The Netherlands, in 1995 and 1999, respectively. In 2000, he joined the Philips Center for Industrial Technology, Eindhoven. In 2007, he joined ASML, Mechatronics Development, Veldhoven, The Netherlands. He was appointed full professor of Industrial nonlinear control for high-precision systems at the Department of Mechanical Engineering at Eindhoven University of Technology (TU/e) on February 1, 2019 for one day a week. His current research interests include the control of industrial motion systems with special focus on nonlinear control, feedforward and learning control, and data-driven optimization. Marcel Heertjes was a recipient of the IEEE Control Systems Technology Award 2015 for variable gain control and its applications to wafer scanners. He acts as an Associate Editor for IFAC Mechatronics since 2016.
Feedback in Wafer Scanners: Use the Unstable
Wafer scanners are complex lithography machines that are critical to the production of integrated circuits (or chips). Driven by the constant need to improve performance in terms of throughput, overlay, focus, and imaging, which can be linked to Moore’s law, the control design of wafer scanners is pushed to its limits. In this context, inherent design limitations in linear feedback control have often provided the motivation for researchers and engineers to explore nonlinear feedback strategies. In the wafer scanner industry, an example is given by the recent developments in hybrid integrator-gain systems, abbreviated with HIGS. HIGS, that operate alternately in so-called integrator mode or in gain mode, have properties and associated (phase) benefits inherited from reset control, in particular the Clegg integrator. However, HIGS do not produce discontinuous control signals due to the absence of (partial) state resets. The latter is considered a favorable property in dealing with structural dynamics of the wafer scanner and the generation of higher harmonics through nonlinear feedback control, especially in the presence of weakly damped resonances. HIGS, and in particular HIGS-PID control, offer the possibility to outperform any linear control, for example in avoiding overshoot, and can benefit from unstable control designs in its underlying modes. The price to pay is increased complexity of the design, stability and performance analysis. In the keynote lecture, an industrial perspective on HIGS-PID control will be sketched that includes the following aspects: (a) inherent design limitations, (b) time- and frequency-domain stability tools, (c) robust nonlinear control design, and (d) lithographic stage applications and performances.
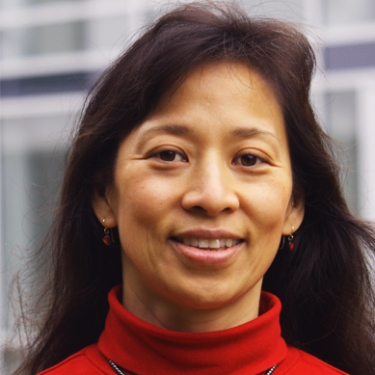
Lucy Pao is a Palmer Endowed Chair Professor in the Electrical, Computer, and Energy Engineering Department at the University of Colorado Boulder. She earned B.S., M.S., and Ph.D. degrees in Electrical Engineering from Stanford University. Her research has primarily focused on engineering control systems, with applications ranging from atomic force microscopes to multi-megawatt wind energy systems. She is a Fellow of the IEEE and IFAC. Selected awards include a National Science Foundation CAREER Award, an Office of Naval Research Young Investigator Award, an IFAC World Congress Young Author Prize, an IEEE Control Systems Magazine Outstanding Paper Award (with K. Johnson), a SIAM Journal on Control and Optimization Best Paper Prize (with J. Marden and H. P. Young), the AACC Control Engineering Practice Award, the European Academy of Wind Energy Scientific Award, and the ASME Nyquist Lecturer Award. Selected past professional society activities include being General Chair of the American Control Conference, an elected member of the IEEE Control Systems Society Board of Governors, Member of the US Defense Science Study Group, and Member of the IFAC Executive Board. Selected current activities include being a Fellow of the Renewable and Sustainable Energy Institute, the Education Liaison on the IFAC Technical Board, and the IFAC Pavel J. Nowacki Distinguished Lecturer.
Control of Floating Offshore Wind Turbines
Wind energy is among the fastest-growing sources of electrical energy worldwide. Compared to land-based wind energy, offshore wind energy has the advantages of increased wind resource availability and consistency, proximity to major population centers, and enabling larger-scale turbines. As such, over the last decade, installed offshore wind power capacity has grown at a phenomenal average annual rate of 33%. Currently, more than 99% of installed offshore wind capacity consists of fixed-bottom wind turbines in shallow waters (<60m deep). Globally, however, 80% of offshore wind resources are at water depths greater than 60m. For such deep waters, floating offshore wind turbines are expected eventually to be as economically competitive as shallow-water fixed-bottom wind turbines. Basic types of floating wind substructures have been derived from platforms used in the oil and gas industry and result in safe but bulky and expensive designs. A novel Ultraflexible Smart FLoating Offshore Wind Turbine (USFLOWT) concept that better optimizes the design of the floating platform may be able to lower the cost of energy for floating offshore wind turbines. This talk will outline how, as part of a large team, we are using a control co-design approach to develop the USFLOWT concept. We describe some of the control challenges and highlight initial controllers and performance results. We close by discussing on-going and future research avenues for the growing floating offshore wind energy area.